Travelling low- and middle-income countries in her twenties made Molly M. Stevens realise how completely unequal the world is in access to resources and healthcare. It made her transform her passion for science into something that could make a difference in people’s lives. By designing nanomaterials to interact with biology, Molly M. Stevens and her team are developing advanced drug delivery systems, ultrasensitive biosensing and tissue engineering scaffolds to recreate eyes, bones and heart. For her groundbreaking research venture into the future of medicine, she is receiving the 2023 Novo Nordisk Prize.
Up to 3.5 billion people – almost half the world’s population – lack access to the health services they need. According to WHO, almost 100 million people each year are being pushed to extreme poverty because of healthcare expenses. Already early in her career, 2023 Novo Nordisk Prize recipient Molly M. Stevens realised that health innovation and digital technologies are some of the keys to solve this inequality.
“I became very motivated to be able to work on science that was not going to just benefit rich people but could also help in democratising access to healthcare. And this is still a huge motivator and helps us to set the challenges within much of the work we do in my team, such as biosensing tests that can detect HIV earlier. By bringing together fundamental concepts around understanding materials and the interface with biology, we aim to develop technologies that can revolutionise access to healthcare,” explains Molly M. Stevens, Professor of Biomedical Materials and Regenerative Medicine from Imperial College London, United Kingdom.
Five years was too long
To someone external who does not know Molly Stevens, it looks like they do many different things, and she says that she is “one of these people” that has always been interested in many different things. In school, she loved geography and languages and sciences. But in the end, she chose science and kept her interest in languages and geography as hobbies. Also, it enabled her to be creative.
"I think science is so creative. The fact that people think it might not be is misinformed. I see lots of parallels between creativity in art and creativity in science. I love exposing myself to lots of art and lots of music, because it is part of my nourishment for keeping an open mind. But science is massively creative. I am a very visual person, so a lot of my creative thinking is in images and thinking about how things come together in three dimensions."
Molly Stevens chose to study pharmacy at Bath University, United Kingdom, because it covered lots of different things – from human biology to chemistry to designing drugs for pharmacology – but not necessarily because she wanted to become a pharmacist.
"I mean, this is ironic, when I think about it now. I had been thinking about studying medicine. And I decided not to, because I thought that 5 years was too long to be at university. I have been there ever since."
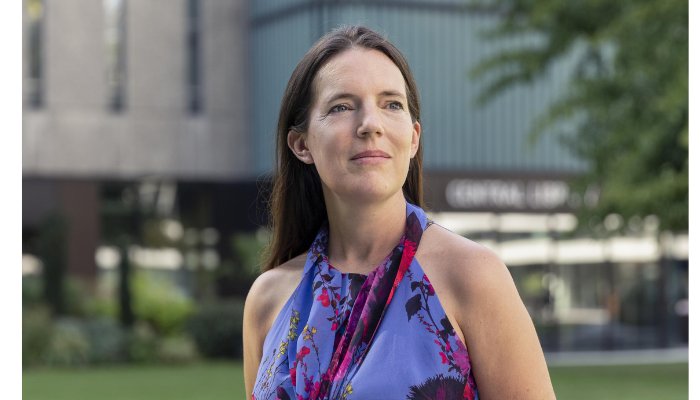
Photo: Molly Stevens.
1000 times better
Bath University is known for a highly regarded student experience and for providing outstanding real-world preparation. And once graduated and after working for a year in hospitals, she decided to discover the real world afterwards by taking time out to go travelling a long time in South-East Asia and India.
“During my travels, I became acutely aware of how completely unequal our world is in people’s access to resources and healthcare. So, ever since, I have always been thinking in the back of my mind: how can we make a difference in this area?”
The time away while travelling also made her wonder what sort of PhD studies she wanted to do.
“I wanted to get my teeth stuck into something. I decided that I wanted to do a PhD that would be as hard as possible. That was my main motivation. I chose single-molecule biophysics because I had not studied physics at A level. And I thought that this sounded difficult and a good challenge.”
For 3 years, Molly Stevens worked with atomic force microscopy – invented in 1985 by IBM scientists – a scanning technique gathering information by touching the surface of a sample with a mechanical probe – a small cantilever. This results in a resolution more than 1000 times better than the optical diffraction limit – enabling scientists to study objects on the order of fractions of a nanometre.
“It was such an exciting time because it was the time where atomic force microscopy was being used for the first time to uncover the binding properties between molecules. And I got to use it to basically unravel peptides that were bound together and to measure the forces between them at the single-molecule level. I became interested in understanding how molecules come together, what holds them together and how can we study this and understand it right down at the nanoscale.”
Later, the studies of biological interactions expanded to investigating protein-to-protein interactions, and they were considered pioneering at the time when atomic force microscopy was emerging. Today, it is one of the foremost tools for imaging, measuring and manipulating matter at the nanoscale.
From a completely different field
The year 2000 in which Molly Stevens finished her PhD studies was full of all sorts of enormous millennium conferences. She attended one in San Francisco, where she happened to be walking past a room where a picture grabbed her attention. On the screen was a little boy with severe liver failure. The talk was about how to design materials that would help the body to heal itself.
“I thought this was just wonderful and it totally, totally inspired me that one’s research could have so much impact on helping people. This was completely unexpected, and within a few minutes I felt certain that this was what I wanted my next field to be.”
After the talk, Molly approached the person giving the talk, and he asked if she could do a postdoctoral fellowship with him – unaware that this was world-renowned engineer Robert Langer.
“I was coming from a completely different field. So, I was probably less nervous speaking with him than I would have been if had realised quite how famous he was, but I just loved his approach and his energy. And, of course, I had a wonderful time in his lab. I kept my skill sets, but I learned to apply them in a completely different way.

Photo: Robert Langer.
The bone bioreactor
The next 3 years in the Department of Chemical Engineering at the MIT in Cambridge, MA developed Molly Stevens’ skills into a more applied direction with an overall goal to affect the lives of people and kickstarted her career in a new field.
“I got to work on an exciting project with Robert Langer and Prasad Shastri – the bone bioreactor – about growing bone on the outside of the legs. So, you could transplant it somewhere else. It seemed like a high-risk project. But I was not too worried about that. I thought it was going to have some chance of success. And if it works, it is going to be amazingly cool.”
Bone is generally quite good at repairing itself in the body. For repairing complex bone fractures, bone grafting from the iliac crest to replace missing bone is the gold standard but can lead to significant pain in the iliac crest years after. The idea with the bone bioreactor was to recreate the generation of bone within the body.
“We have a layer of stem cells along our long bones called the periosteum. My contribution to this was developing liquid that we would inject under this layer. The liquid turn into a rigid gel to create an artificial cavity next to the bone, and it worked out. These stem cells wake up when you have a fracture, and we managed to create the same effect with liquid, so we were able to generate amazing amounts of well-organised bone for transplantation elsewhere in the body.”
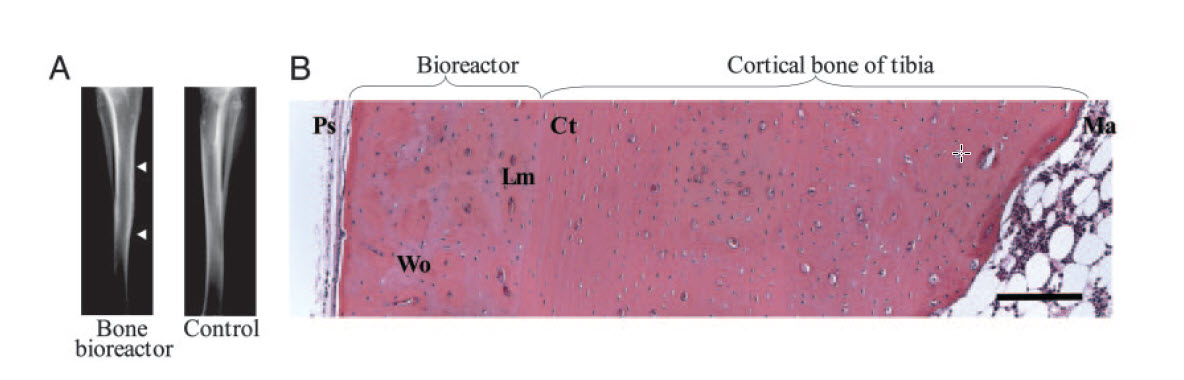
Photo: The researchers managed to generate amazing amounts of well-organised bone for transplantation elsewhere in the body.
A feeling of positive energy
A simple alginate gel – derived from seaweed – formed the scaffold for the bone bioreactor. Likewise, Molly Stevens' stay at MIT turned out to form part of the crucial foundation for her future career. Following her PhD, in 2004, she joined Imperial College London, where she then started a highly interdisciplinary research group.
The team and a palpable feeling of positive energy is a key to success. You have biologists, engineers, physicists, material scientists and surgeons. It is amazing. Because if you have a group meeting, you can be incredibly innovative and bounce a lot of ideas around and get a healthy dose of realism, because we might propose something from an engineering viewpoint. And the surgeon might just turn around and say that we do not do that in practice.
People often told Molly that she should either focus on completely fundamental research or very applied research. But she refused to listen because it did not make sense.
Because the insights you get from understanding something, for example, at the nanoscale, can feed directly into making the best applied innovations.

Photo: Imperial College London
Capturing the degree of complexity
Again and again during her career, Molly Stevens has thus returned to the starting-point: learning the basics through thorough analysis. One method that has stuck with her is Raman spectroscopy – a chemical analysis technique based on the interaction of light with the chemical bonds within a material. The technique is both non-destructive to living material and delivers detailed information such as crystallinity, structure and molecular interactions – and can thus help provide a biochemical fingerprint of a living cell.
“I am interested in how cells interact with materials: for example, to help in tissue regeneration and organ regeneration. You might design new materials by simply mimicking the bulk properties of an existing one, but it makes a lot of sense to also think and examine what is happening right down at the nanoscale or at the level of the cell, so that you can recreate materials that are going to give the best possible cell environment to make the best possible tissue.”
Deciding when to divide, when to specialise and when to self-destruct are ongoing processes within cells, but equally important to regulate cell fate are external signals from the surrounding extracellular matrix.
“Bone, for example, is beautifully organised with lots of bloods vessels and a 3D matrix of equally organised nanofibres that give a lot of information to the cells – an extracellular matrix that is part organic and part inorganic. A key challenge of our work has thus been to capture the degree of complexity that is needed to functionally replicate the natural tissues as complex as bone, cartilage, heart and the eye.”
In very different new directions
Whereas some tissues like bone can be grown in situ in a bone bioreactor, Molly Stevens quickly realised that other kinds need other scaffolds. Already in 2007, she had co-founded a company that developed platforms to grow and repair both soft and hard tissue based on her research, and she served as Chief Scientific Officer in the company for 5 years in parallel with her academic positions.
We wanted to have enough information to get the cells to do what we want but not be so complex that it would make it too difficult to get to the clinic. Only by understanding the fundamental concepts around materials and the interface with materials can we solve some of the key problems. And one thing we thought about a lot at that time was trying to understand the structure of the tissue in the body.
In 2008, Molly Stevens was promoted to Professor, making her one of the youngest professors in the history of Imperial College London. The applied work Molly did there in the beginning was still in regenerative medicine, leading on from some of the things she learned during her postdoctoral fellowship but characteristically also went in new and very different directions.
“To someone external, it would look like we do many different things: advanced delivery systems, ultrasensitive biosensing and tissue engineering scaffolds. But to me, they are all the same thing. They are designing materials to interact in interesting ways with biology. And that is the sort of central foundation for all these different areas where we apply this insight.”
Identifying marginal cells of a tumour
Molly Stevens envisaged how the work on materials could lead to medical implants engineered with nanoscale features to enable stronger interaction with the host tissue for a longer time but also how the nanostructures could lead to both implanted biosensors and bioactive composites to deliver drugs.
One of our great motivations has been that we have been using different types of nanoparticles for drug delivery or maybe in trying to make new and better vaccines. We have also worked in collaboration with the Houston Methodist Research Institute on materials that look like a tiny bit of needles. Cells can interact with them, and you might get 50 or more of those needles per cell. And what that can do is help you to deliver things into cells and tissues but also monitor things that are happening within those cells or tissues.
The researchers showed arrays of biodegradable and biocompatible needles could be fabricated using chemical etching and standard microfabrication. The needles – created out of many different materials and only nanometres at the apex – could be used to deliver nucleic acids such as DNA or proteins to cells but could also be used for intracellular sensing of biomarkers in a highly efficient and non-toxic way.
“We were interested in how we could perhaps measure differences between cancer cells and healthy cells. We know that there are many differences in the environment – pH and in intracellular enzymatic activity – especially the protease called cathepsin B, which is upregulated in cancer cells, and so we functionalised the needles with short peptides that had a fluorophore on the end.”
A fluorophore is a chemical compound that can re-emit light when excited by light. When the cathepsin B-protease in the cancer cells cleaves the fluorophore, it lights up – which enables surgeons to identify marginal cells of a tumour and to remove it completely with higher precision. The researchers have also been able to use the nanoneedles to deliver quantum dots – nanocrystals of a semiconducting material – into the cells.
I was excited about this since our group was the first to use these quantum dots for measuring enzyme activities such as kinase and acetyl transferase enzymes.
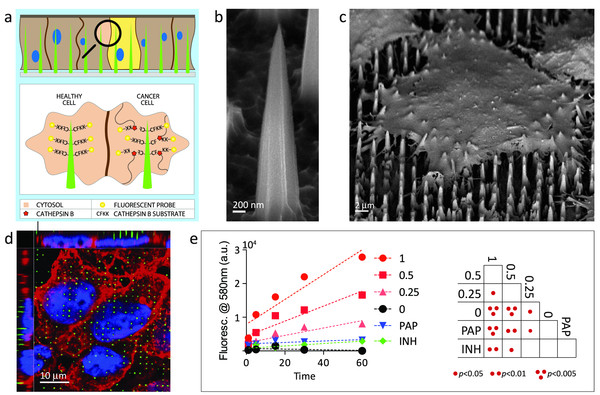
Photo: The needles – created out of many different materials and only nanometres at the apex – could be used to measure differences between cancer cells and healthy cells.
Turning things upside down
The journey of Molly Stevens’ team into understanding the interface between materials and biology has thus led to major advances in both regenerative medicine and how nanoparticles can target and deliver cargoes in the body. Sensors for detecting disease is the third and equally large part of the research portfolio of the Stevens Group at Imperial College London.
“The work has key obvious applications within infectious diseases but also for detecting early heart failure or cancer. All these diseases can benefit from early detection, and this drives us. How can we use these nanomaterial systems to enable better early detection so that our patients can ultimately be treated better?”
The work focuses on precluding the need for complex and expensive instrumentation and instead designing and developing systems that can be read out with the naked eye. In some of her earlier work, Molly and her colleagues introduced a system that could detect ultralow concentrations of prostate-specific antigen in serum.
To do this, the researchers took nanometre-sized gold particles that were linked together via short chains of amino acid at their surface. When the nanoparticles are aggregated, they form a blue solution. But when the solution contains the prostate-specific antigen enzyme – related to prostate cancer – the link is cut, the particles separate and the solution turns red. And because each enzyme can cut through many molecules, again the signal is amplified. This out-of-the-box thinking is characteristic of Molly’s research.
I think many of the ideas come from having different disciplines coming together. The interface between different disciplines is a challenging thing, but it is so exciting, because it is less studied in many ways than the individual disciplines. You can have a lot of creativity at these interfaces.
A plethora of applications
The invention of new and sometimes surprising concepts is characteristic of Molly Stevens’. A recent example of this is her group’s exploitation of buoyancy principles to generate new materials bearing well-defined compositional, mechanical or biochemical gradients.
The tissues and bones that make up our bodies are complex and formed from a variety of components. Replicating these materials has therefore been complicated. We therefore tried to develop a simple, quick and versatile way of creating them.
The targeted challenge was the osteochondral interface – the region between cartilage and bone.
“It is an inhomogeneous construct with a natural gradient across multiple materials. By injecting one liquid into a second denser one, we were able to produce a tuneable gradient simply by exploiting the fundamental force of buoyancy. We could then preserve the gradients by gelation and polymerisation.”
Molly’s team took things even further to form gradients with various nanoparticles and small molecules, and they were even able to tune sharp and gradient transitions in the material.
This opens up possibilities for a plethora of applications in gradient material fabrication and interfacial tissue engineering.

Photo: By injecting one liquid into a second denser one, we were able to produce a tuneable gradient simply by exploiting the fundamental force of buoyancy.
Enabling the design of better medicines
However, one thing had so far kept Molly Stevens’ team from catalysing the biggest revolutions.
“One of our great motivations has been using different types of nanoparticles to try to make new and better therapeutics, but one frustration we had is the lack of techniques that could help you to understand nanoparticles at the single-particle level.”
For nanoparticles in particular, heterogeneity in the material can severely affect function and applicability, and conventional chemical analysis techniques only worked for whole big population of nanoparticles.
“Without more fundamental understanding, we are more limited in rational design that can help us with applications for all sorts of things. What my team has done is invent a technique that is the first in the world that can trap single nanoparticles and measure their chemistry in an automated way.
With SPARTA® (Single Particle Automated Raman Trapping Analysis), the team used their experience using Raman-based characterisation to develop a machine that automatically can trap particles and analyse their chemistry for size, composition, reactivity and surface functionalisation.
“That can have many different important applications. You could understand how much drug is inside a material. How are proteins associating to the shell of the nanoparticle? And how does this affect how a particle travels in the body and gets to the right cells of interest? With our technology, you can now measure real-time changes on a single particle, which is just amazing to me.”
SPARTA® is already helping researchers to study other biological and nano-bio systems.
It can enable you to design better medicines. You can monitor the quality of nanoparticle products better, but you can also make improvements to the design as well.
A dream comes true
Molly Stevens has just been appointed to the John Black Professorship between Medicine and Engineering at the University of Oxford and will be Deputy Director of the newly established interdisciplinary Kavli Institute for NanoScience Discovery. But even though her career has been extremely successful, one dream still lurks in the back of her mind: the dream that originally ignited her desire to revolutionise the medical world.
“When you are traveling, for example in Africa, you see a lot of people in difficult conditions. We are just so privileged in high-income economies. That has always been very motivating for me, and I always keep that in the back of my mind. My dream is for our science to help people all around the world.”
Worldwide, many infections remain undiagnosed and untreated because of poor diagnostic tools, resulting in ongoing transmission of serious infections or delay in identifying emerging threats, leading to major consequences for millions of people. For example, an estimated 38 million people are currently living with HIV.
“The existing tests detected antibodies to HIV, which means that you must wait weeks, and that is because the tests that could be used in the field to detect the virus itself were not sensitive enough.“

Photo: An estimated 38 million people are currently living with HIV
Democratising worldwide access
To solve this, the researchers transformed the existing lateral flow assay – like COVID-19-quick tests – to sense p24 – a protein found on the outside of the HIV virus. By incorporating in-house designed catalytic nanoparticles that produced an amplification stage, they increased the sensitivity up to 100-fold, enabling detection even when virus level is very low.
The plan is to distribute and use the tests in remote settings: a plan taking advantage of the current expansion in mobile phone technology, with an estimated 6 billion users worldwide.
Many people will have difficulty in getting to a healthcare centre, but they could use a test that could be read by their own mobile phone. By making a test that can work in decentralised settings, you can access more people, and again, help to democratise access to healthcare.
Molly Stevens believes that having these kinds of simple and robust tests with ultrasensitive biosensing capability can help ease the effects of many infectious diseases that affect people all around the world, as seen with COVID-19.
“Early diagnosis plays a vital role in the treatment, care and prevention of infectious diseases, but especially based on the impact in low- and middle-income countries, there is a great need for diagnostic tests that can detect diseases much earlier at affordable price points. My hope is that when this succeeds, it can finally pave the way towards democratised worldwide access to advanced healthcare technologies.”